Modern trends in
Page 60
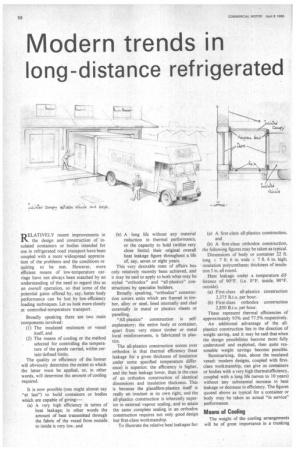
Page 61
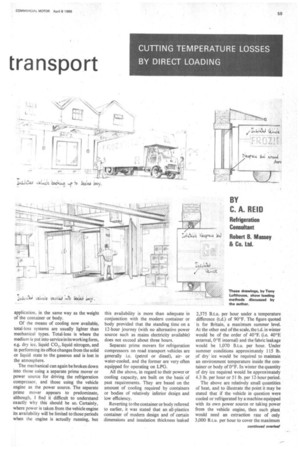
Page 62
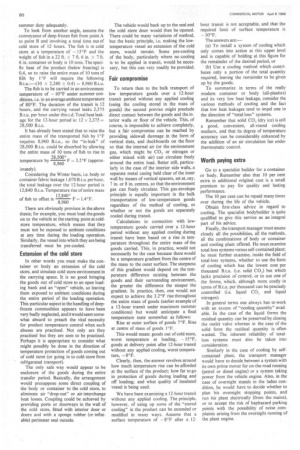
If you've noticed an error in this article please click here to report it so we can fix it.
long-distance refrigerated transport
RELATIVELY recent improvements in the design and construction of insulated containers or bodies intended for use in refrigerated road transport have been coupled with a more widespread appreciation of the problems and the conditions requiring to be met. However, more efficient means of low-temperature carriage have not always been matched by an understanding of the need to regard this as an overall operation, so that some of the potential gains offered by. say, better body performance can be lost by low-efficiency loading techniques. Let us look more closely at controlled-temperature transport.
Broadly speaking there are two main components involved: (I) The insulated enclosure or vessel itself, and
(2) The means of cooling or the method selected for controlling the temperature of the goods carried, within certain-defined limits.
The quality or efficiency of the former will obviously determine the extent to which the latter must be applied, or, in other words, will determine the amount of cooling required.
It is now possible (one might almost say "at last") to build containers or bodies which are capable of giving:—
(a) A very high efficiency in terms of heat leakage; in other words the amount of heat transmitted through the fabric of the vessel from outside , to inside is very low, and (b) A long life without any material reduction in thermal performance, or the capacity to hold (within very close limits) their original overall heat leakage figure throughout a life of, say, seven or eight years.
This very desirable state of affairs has only relatively recently been achieved, and it may be said to apply to both what may be styled "orthodox" and "all-plastics" constructions by specialist builders.
Broadly speaking, "orthodox" construction covers units which are framed in timber, alloy or steel, lined internally and clad externally in metal or plastics sheets or panelling.
"All-plastics" construction is selfexplanatory; the entire body or container, apart from very minor timber or metal local reinforcements, is fabricated in plastics.
The all-plastics construction scores over orthodox in that thermal efficiency (heat leakage for a given thickness of insulation under some specified temperature difference) is superior; the efficiency is higher, and the heat leakage lower, than in the case of an orthodox construction of identical dimensions and insulation thickness. This is because the glassfibre-plastics itself is really an insulant in its own right, and the all-plastics construction is inherently superior in external vapour sealing, and to attain the same complete sealing in an orthodox construction requires not only good design but first-class workmanship.
To illustrate the relative heat leakages for: (a) A first-class all-plastics construction. and (b) A first-class orthodox construction, the following figures may be taken as typical.
Dimensions of body or container 22 ft. long x 7 ft. 6 in. wide x 7 ft. 6 in. high; insulation polyurethane; thickness of insulation 5 in. all round.
Heat leakage under a temperature difference of 90°F. (i.e. 0°F. inside, 90°F. outside).
(a) First-class all-plastics construction 2,375 B.t.u. per hour.
(b) First-class orthodox construction 2,850 B.t.u. per hour.
These represent thermal efficiencies of approximately 93% and 77.5% respectively.
An additional advantage of the allplastics construction lies in the direction of weight saving, and it may be said that when the design possibilities become more fully understood and exploited, then quite reasonable weight savings become possible.
Summarizing, then, about the insulated vessel: modern designs, coupled with firstclass workmanship, can give us containers or bodies with a very high thermal efficiency, coupled with a long life (seven to 10 years) without any substantial increase in heat leakage or decrease in efficiency. The figures quoted above as typical for a container or body may be taken as actual "in service" performance.
Means of Cooling
The weight of the cooling arrangements will be of great importance in a trunking application, in the same way as the weight of the container or body.
Of the means of cooling now available, total-loss systems are usually lighter than mechanical types. Total-loss is where the medium is put into service inits working form, e.g. dry ice, liquid CO„ liquid nitrogen, and in performing its office changes from the solid or liquid state to the gaseous and is lost in the atmosphere.
The mechanical can again be broken down into those using a separate prime mover or power source for driving the refrigeration compressor, and those using the vehicle engine as the power source. The separate prime mover appears to predominate, although, I find it difficult to understand exactly why this should be so. Certainly, where power is taken from the vehicle engine its availability will be limited to those periods when the engine is actually running, but this availability is more than adequate in conjunction with the modern container or body provided that the standing time on a 12-hour journey (with no alternative power source such as mains electricity available) does not exceed about three hours.
Separate prime movers for refrigeration compressors on road transport vehicles are generally i.e. (petrol or diesel), airor water-cooled. and the former are very often equipped for operating on LPG.
All the above, in regard to their power or cooling capacity, are built on the basis' of past requirements. They are based on the amount of cooling required by containers or bodies of relatively inferior design and low efficiency.
Reverting to the container or body referred to earlier, it was stated that an all-plastics container of modern design and of certain dimensions and insulation thickness leaked 2,375 B.t.u. per hour under a temperature difference (t.d.) of 90°F. The figure quoted is for Britain, a maximum summer level. At the other end of the scale, the t.d. in winter would be of the order of 40°F. (i.e. 40°E external, 0°F internal) and the fabric leakage would be 1,070 B.t.u. per hour. Under summer conditions approximately 115 lb. of dry' ice would be required to maintain an environment temperature inside the container or body of 0°F. In winter the quantity of dry ice required would be approximately 4.3 lb. per hour or 51 lb. per 12-hour period.
The above are relatively small quantities of heat, and to illustrate the point it may be stated that if the vehicle in question were cooled or refrigerated by a machine equipped with its own power source or taking power from the vehicle engine, then such plant would need an extraction rate of only 3,000 B.t.u. per hour to cover the maximum summer duty adequately.
To look from another angle, assume the conveyance of deep-frozen fish from point A to point B and involving a total time out of cold store of 12 hours. The fish is in cold store at a temperature of —15°F and the weight of fish in a 22 ft. x 7 ft. 6 in. x 7 ft. 6 in. container or body is 10 tons. The specific heat of the product (below freezing) is 0.4, so to raise the entire mass of 10 tons of ah by 1°F will require the following B.t.u.:—(10 x 2,240 X 0.4) = 8,960 B.t.u.
The fish is to be carried in an environment temperature of —10°F under summer conditions, i.e. in an average ambienttemperature of 80°F. The duration of the transit is 12 hours, and the carrying vessel leaks 2,375 B.t.u. per hour under this t.d. Total heat leakage for the 12-hour period is: 12 x 2.375 = 28,500 B.t.u.
It has already been stated that to raise the entire mass of the transported fish by 1°F requires 8,960 B.t.u., so the "in-leak" of 28,500 B.t.u. could be absorbed by allowing the entire mass of IQ tons of fish to rise in 28,960 500° 8, 28,960 500° 8, temperature by — F = 3.2°F (approximately).
Considering the Winter basis, i.e. body or container fabric leakage 1,070 B.t.u. per hour, the total leakage over the 12-hour period is 12,840 B.t.u. Temperature rise of entire mass 12,840° of fish to offset is — 960 F = 1.4°F.
8,
There are obviously provisos in the above thesis; for example, you must load the goods on to the vehicle at the starting point at coldstore temperature, which means that they must not be exposed to ambient conditions at any time during the loading operation. Similarly, the vessel into which they are being transferred must be pre-cooled.
Extension of the cold store
In other words you must make the container or body an extension of the cold store, and simulate cold store environment in the carrying space. It is no good bringing the goods out of cold store to an open loading bank and an "open" vehicle, so leaving them exposed to ambient conditions during the entire period of the loading operation. This particular asfiect in the handling of deepfrozen commodities appears to have been very badly neglected, and it would seem somewhat absurd to preach the vital necessity for product temperature control when such abuses are practised. Not only are they practised but they are seen to be practised. Perhaps it is appropriate to consider what might possibly be done in the direction of temperature protection of goods coming out of cold store (or going in to cold store from refrigerated transport).
The only safe way would appear to be enclosure of the goods during the entire transfer period. Basically, the arrangement would presuppose some direct coupling of the body or container to the cold store, to eliminate air "drop-out" or air-interchange heat losses. Coupling could be achieved by providing ports or doorways in the wall of • the cold store, fitted with interior door or doors and with a sponge rubber (or inflatable) perimeter seal outside. The vehicle would back up to the seal and the cold store door would then be opened. There could be many variations of method, but the basic principle, i.e. making the lowtemperature vessel an extension of the cold store, would remain. Some pre-cooling of the body, particularly where no cooling is to be applied in transit, would be necessary, but this can very readily be provided.
Fair compromise
To return then to the bulk transport of low temperature goods over a 12-hour transit period without any applied cooling (using the cooling stored in the mass of goods) the second proviso might preclude direct contact between the goods and the interior walls or floor of the vehicle. This, of course, is impossible to achieve completely, but a fair compromise can be reached by providing sidewall dunnage in the form of vertical slats, and duckboards on the floor so that the internal air (or the environment gas, which might be CO, or nitrogen or either mixed with air) can circulate freely around the entire load. Better still, particularly in the case of the interior side walls, a separate metal casing held clear of the inner wall by means of vertical spacers, set at, say, 7 in. or 8 in. centres, so that the environment gas can freely circulate. This gas-envelope principle is equally important in the bulk transportation of low-temperature goods regardless of the method of cooling, or whether or not the goods are separately cooled during transit.
Calculations in connection with lowtemperature goods carried over a 12-hour period without any applied cooling during transit have been based on a rise in temperature throughout the entire mass of the goods carried. This, in practice, would not necessarily be the case because there would be a temperature gradient from the centre of the mass to the outer surface. The steepness of this gradient would depend on the temperature difference existing between the goods and their surrounding environment, the greater the difference the steeper the gradient. In practice, then, one would not expect to achieve the 3.2°F rise throughout the entire mass of goods (earlier example of a 12-hour transit under maximum summer conditions) but would anticipate a final temperature state somewhat as follows: Rise at outer surface of goods 7°F. Rise at centre of mass of goods 1°F.
This would mean: goods out of cold store, worst temperature at loading, — 15°F, goods at delivery point after 12-hour transit without any applied cooling, worst temperature, —8°F.
Clearly, then, the answer revolves around how much temperature rise can be afforded at the surface of the product; how far to go in protection of goods during loading and off loading; and what quality of insulated vessel is being used.
We have been examining a 12-hour transit without any applied cooling. The principle, however, of using up some of the "stored cooling" in the product can be extended or modified in many ways. Assume that a surface temperature of —8°F after a 12 hour transit is not acceptable, and that the required limit of surface temperature is —10°F.
The answers are:— (a) To install a system of .cooling which only comes into action at this upper level and is capable of holding at this figure for the remainder of the desired period, or
(b) Use a cooling method which .contributes only a portion of the total quantity required, leaving the remainder to be given up by the goods.
To summarize in terms of the really modern container or body (all-plastics) and its very low heat leakage, consider the various methods of cooling and the fact that low heat leakages tend to impel one in the direction of "total-loss" systems.
Remember that solid CO, (dry ice) is still a good, convenient, all-round cooling medium, and that its degree of temperature accuracy can be considerably enhanced by the addition of an air circulation fan under thermostatic control.
Worth paying extra
Go to a specialist builder for a container or body. Remember also that 10 per cent extra in additional original cost is a small premium to pay for quality and lasting performance.
The 10 per cent can be repaid many times over during the life of the vehicle.
Obtain first-class advice in regard to cooling. The specialist bodybuilder is quite qualified to give this service as an integral part of his advice.
Finally, the transport manager must assess closely all the possibilities, all the methods, all the combinations of body or container and cooling plant offered. He must examine total-loss systems versus self-contained plant; he must further examine, inside the field of total-loss systems, whether to use the form of cooling which has the lowest cost per thousand B.t.u. (i.e. solid CO,) but which lacks precision of control, or to use one of the forms, which, although more costly in terms of B.t.u. per thousand can be precisely controlled (i.e. liquid CO, and liquid nitrogen).
In general terms one always has to work with an excess of "cooling quantity" available. In the case of the liquid forms the residual quantity can be preserved by closing the outlet valve whereas in the case of the solid form the residual quantity is often wasted. The inherent reliability of totalloss systems must also be taken into consideration.
Similarly in the case of cooling by selfcontained plant, the transport manager would have to decide between a system with its own prime mover for on-the-road running (petrol or diesel engine) or a system taking power from the vehicle engine. Also, in the case of overnight stands in the laden condition. he would have to decide whether to plan his overnight stopping points, and run his plant electrically (from the mains), or to accept the risk of haphazard parking points with the possibility of noise complaints arising from the overnight running of the plant engine.