Gas turbines for trucks: a realistic assessment of potential
Page 226
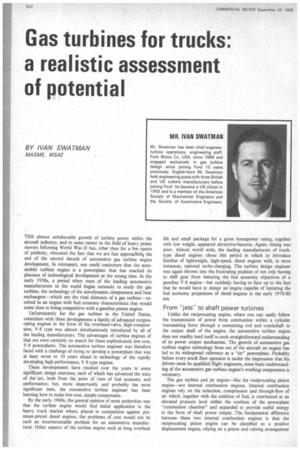
Page 227
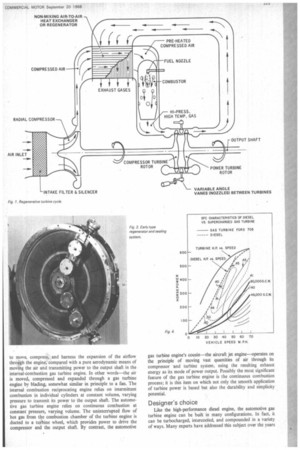
Page 228
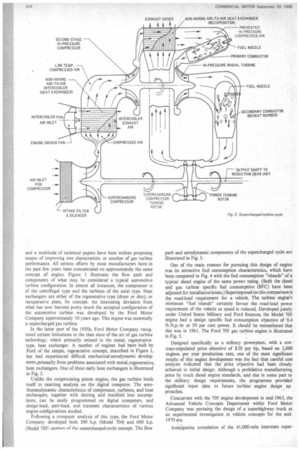
Page 231
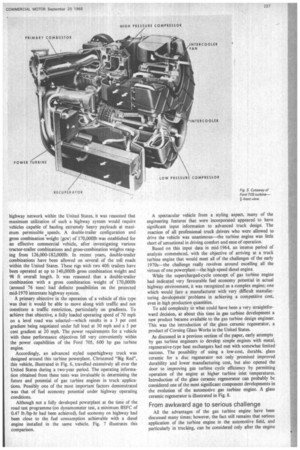
Page 233
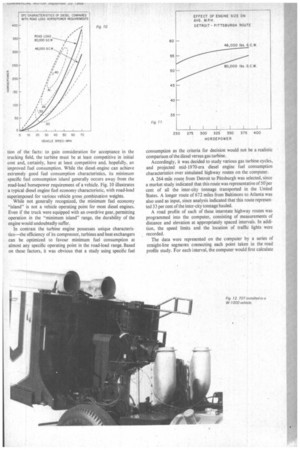
Page 234
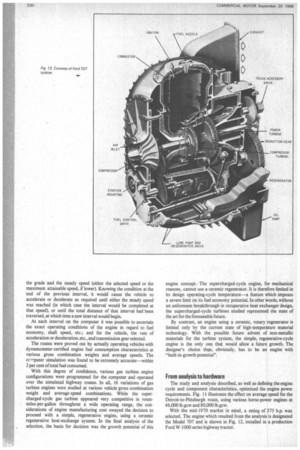
Page 237
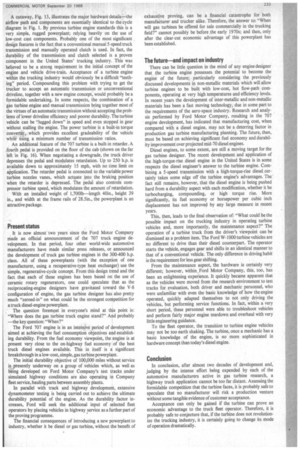
If you've noticed an error in this article please click here to report it so we can fix it.
BY IVAN SWATMAN
MASME, MSAE
THE almost unbelievable growth of turbine power within the aircraft industry, and to some extent in the field of heavy prime movers following World War. It has, other than for a few spurts of publicity, obscured the fact that we are fast approadhifig the end of the second decade of automotive gas turbine engine development. In retrospect, one could conjecture that the automobile turbine engine is a powerplant that has reached its plateaus of technological development at the wrong time. In the early 1950s, a period when most of the leading automotive manufacturers in the world began seriously to study the gas turbine, the technology of the aerodynamic components and heat exchangers—which are the vital elements of a gas turbine—resulted in an engine with fuel economy characteristics that would come close to being competitive with a gasoline piston engine.
Lhfortunately for the gas turbine in the United States, coincident with these developments a family of advanced reciprocating engines in the form of the overhead-valve, high-compression, V-8 type was almost simultaneously introduced by all of the leading manufacturers. The advantages of turbine engines of that era were certainly no match for these sophisticated. low-cost, V-8 powerplants. The automotive turbine engineer was therefore faced with a challenge of trying to develop a powerplant that was at least seven to 10 years ahead in technology of the rapidly developing high-performance, V-8 type engines.
These developments have resulted over the years in some significant design exercises, each of which has advanced the state of the art, both from the point of view of fuel economy and performance; but, more importantly and probably the most significant item, the automotive turbine engineer has been learning how to make low-cost, simple components.
By the early 1960s, the general opinion of most authorities was that the turbine engine would find initial application in the heavy truck market where, placed in competition against premium-priced diesel engines, the problems of cost would not be such an insurmountable problem for an automotive manufacturer. Other aspects of the turbine engine such as long overhaul life and small package for a given horsepower rating, together with low weight, appeared attractive•features. Again, timing was poor. Almost world wide, the leading manufacturers of trucktype diesel engines chose this period in which to introduce families of lightweight, high-speed, diesel engines with, in most instances, optional turbo-charging. The turbine design engineer was again thrown into the frustrating position of not only having to shift gear from bettering the fuel economy objectives of a gasoline V-8 engine—but suddenly having to face up to the fact that he would have to design an engine capable of bettering the fuel economy projections of diesel engines in the early 1970-80 era.
From "jets" to shaft power turbines
Unlike the reciprocating engine, where one can easily follow the transmission of power from combustion within a cylinder transmitting force through a connecting rod and crankshaft to the output shaft of the engine, the automotive turbine engine does not readily lend itself to such straightforward understanding of its power output mechanism. The growth of automotive gas turbine engine technology from out of the aircraft jet engine has led to its widespread reference as a "jet" powerplant. Probably before every truck fleet operator is under the impression that his drivers must be qualified flight engineers, some basic understanding of the automotive gas turbine engine's working components is necessary.
The gas turbine and jet engine—like the reciprocating piston engine—are internal combustion engines. Internal combustion engines rely on the induction, compression and through-flow of air which, together with the addition of fuel, is corn busted at an elevated pressure level within the confines of the powerplant "combustion chamber" and expanded to provide useful energy in the form of shaft power output. The fundamental difference between these two internal combustion engines is that the reciprocating piston engine can be classified as a positive displacement engine, relying on a piston and valving arrangement
to move, compress, and harness the expansion of the airflow through the engine, compared with a pure aerodynamic means of moving the air and transmitting power to the output shaft in the internal-combustion gas turbine engine. In other words—the air is moved, compressed and expanded through a gas turbine engine by blading, somewhat similar in principle to a fan. The internal combustion reciprocating engine relies on intermittent combustion in individual cylinders at constant volume, varying pressure to transmit its power to the output shaft. The automotive gas turbine engine relies on continuous combustion at constant pressure, varying volume. The uninterrupted flow of hot gas from the combustion chamber of the turbine engine is ducted to a turbine wheel, which provides power to drive the compressor and the output shaft. By contrast, the automotive
gas turbine engine's cousin—the aircraft jet engine—operates on the principle of moving vast quantities of air through its compressor and turbine system. using the resulting exhaust energy as its mode of power output. Possibly the most significant feature of the gas turbine engine is the continuous combustion process; it is this item on which not only the smooth application of turbine power is based but also the durability and simplicity potential.
Designer's choice
Like the high-performance diesel engine, the automotive gas turbine engine can be built in many configurations. In fact, it can be turbocharged, intercooled, and compounded in a variety of ways. Many experts have addressed this subject over the years and a multitude of technical papers have been written proposing means of improving one characteristic or another of gas turbine performance. All serious efforts by most manufacturers have in the past few years been concentrated on approximately the same concept of engine. Figure 1 illustrates the flow path and components of what may be considered a typical automotive turbine configuration. In almost all instances, the compressor is of the centrifugal type and the turbines of the axial type. Heat exchangers are either of the regenerative type (drum or disc), or recuperative plate, fin concept. An interesting deviation from what has now become pretty much the accepted configuration of the automotive turbine was developed by the Ford Motor Company approximately 10 years ago. This engine was essentially a supercharged gas turbine.
In the latter part of the 1950s, Ford Motor Company recognized certain limitations in the then state of the art of gas turbine technology, which primarily existed in the metal, regenerativetype. heat exchanger. A number of engines had been built by Ford of the simple, regenerative concept, described in Figure I, but had experienced difficult mechanical-aerodynamic develop ment, primarily from problems associated with metal, regenerative, heat exchangers, One of these early heat exchangers is illustrated in Fig. 2.
Unlike the reciprocating piston engine, the gas turbine lends itself to exacting analysis on the digital computer. The aerothermodynamic characteristics of compressor, turbines, and heat exchangers, together with ducting and manifold loss assumptions, can be easily programmed on digital computers, and design-load, part-load, and transient characteristics of various engine configurations studied.
Following a computer analysis of this type, the Ford Motor Company developed both 300 h.p. (Model 704) and 600 h.p. (Model 705) enctineq of the supercharged-cycle concept. The flow
path and aerodynamic components of the supercharged cycle are illustrated in Fig. 3.
One of the main reasons for pursuing this design of engine was its attractive fuel consumption characteristics, which have been compared in Fig. 4 with the fuel consumption "islands" of a typical diesel engine of the same power rating. (Both the diesel and gas turbine specific fuel consumption (SFC) have been adjusted for installation losses.) Superimposed on this comparison is the road-load requirement for a vehicle. The turbine engine's minimum "fuel islands" certainly favour the road-load power requirement of the vehicle as speed is reduced. Developed jointly under United States Military and Ford finances, the Model 705 engine had a design specific fuel consumption objective of 0.4 lb/h.p-hr at 50 per cent power. It should be remembered that this was in 1961. The Ford 705 gas turbine engine is illustrated in Fig. 5.
Designed specifically as a military powerplant, with a contract-stipulated price objective of $20 per hp, based on 1,000 engines per year production rate, one of the most significant results of this engine development was the fact that careful cost analysis indicated that the price objective had been closely achieved in initial design. Although a prohibitive manufacturing price by truck diesel engine standards, and due in some part to the .military design requirements, the programme provided significant input data to future turbine engine design approaches.
Concurrent with the 705 engine development in mid-1963, the Advanced Vehicle Concepts Department within Ford Motor Company was pursuing the design of a superhighway truck as an experimental investigation in vehicle concepts for the mid1970 era.
Anticipating completion of the 41,000-mile interstate super highway network within the United States, it was reasoned that maximum utilization of such a highway system would require vehicles capable of hauling extremely heavy payloads at maximum permissible speeds. A double-trailer configuration and gross combination weight (gcw) of 170,000lb was established for an effective commercial vehicle, after investigating various tractor-trailer combinations and gross-combination weights ranging from 126,000-182,000lb. In recent years, double-trailer combinations have been allowed on several of the toll roads within the United States. These rigs with two 40ft trailers have been operated at up to 140,0001b gross combination weight and 98 ft overall length. It was reasoned that a double-trailer combination with a gross combination weight of 170,0001b (around 76 tons) had definite possibilities on the projected mid-1970 interstate highway system.
A primary objective in the operation of a vehicle of this type was that it would be able to move along with traffic and not constitute a traffic restriction, particularly on gradients. To achieve that objective, a fully loaded operating speed of 70 mph on a level road was selected—which results in a 3 per cent gradient being negotiated under full load at 30 mph and a 5 per cent gradient at 20 mph. The power requirements for a vehicle with these performance objectives fell very conveniently within the power capabilities of the Ford 705, 600 hp gas turbine engine.
Accordingly, an advanced styled superhighway truck was designed around this turbine powerplant. Christened "Big Red", this vehicle, illustrated in Fig. 6, travelled extensively all over the United States during a two-year period. The operating information obtained from these tests was invaluable in determining the future and potential of gas turbine engines in truck applications. Possibly one of the most important factors demonstrated was that of fuel economy potential under highway operating conditions.
Although not a fully developed powerplant at the time of the road test programme (on dynamometer test, a minimum BSFC of 0.47 lb/hp-hr had been achieved), fuel economy on highway had been close to the fuel consumption achievable with a diesel engine installed in the same vehicle. Fig. 7 illustrates this comparison. A spectacular vehicle from a styling aspect, many of the engineering features that were incorporated appeared to have significant input information to advanced truck design. The reaction of all professional truck drivers who were allowed to drive the vehicle was unanimous—the turbine engine was little short of sensational in driving comfort and ease of operation.
Based on this input data in mid-1964, an intense period of analysis commenced, with the objective of arriving at a truck turbine engine that would meet all of the challenges of the early 1970s—the challenge really revolves around excelling all the virtues of one powerplant—the high-speed diesel engine.
While the supercharged-cycle concept of gas turbine engine had indicated very favourable fuel economy potential in actual highway environment, it was recognized as a complex engine; one which would face a manufacturer with very difficult manufacturing development problems in achieving a competitive cost, even in high production quantities.
To add complexity to what could have been a very straightforward decision, at about this time in gas turbine development a new product became available to the gas turbine design engineer. This was the introduction of the glass ceramic regenerator, a product of Corning Glass Works in the United States.
As discussed in a previous section of the paper, early attempts by gas turbine engineers to develop simple engines with metal, regenerative-type heat exchangers had met with somewhat limited success. The possibility of using a low-cost, durable, glass ceramic for a disc regenerator not only promised improved durability and lower manufacturing cost, but also opened the door to improving gas turbine cycle efficiency by permitting operation of the engine at higher turbine inlet temperatures. Introduction of the glass ceramic regenerator can probably be considered one of the most significant component developments in the evolution of the automotive gas turbine engine. A glass ceramic regenerator is illustrated in Fig. 8.
From awkward age to serious challenge
All the advantages of the gas turbine engine have been discussed many times; however, the fact still remains that serious application of the turbine engine in the automotive field, and particularly in trucking, can be considered only after the engine tion of the facts: to gain consideration for acceptance in the trucking field, the turbine must be at least competitive in initial cost and, certainly, have at least competitive and, hopefully, an improved fuel consumption. While the diesel engine can achieve extremely good fuel consumption characteristics, its minimum specific fuel consumption island generally occurs away from the road-load horsepower requirement of a vehicle. Fig. 10 illustrates a typical diesel engine fuel economy characteristic, with road-load superimposed for various vehicle gross combination weights.
While not generally recognized, the minimum fuel economy "island" is not a vehicle operating point for most diesel engines. Even if the truck were equipped with an overdrive gear, permitting operation in the "minimum island" range, the durability of the engine would undoubtedly suffer.
In contrast the turbine engine possesses unique characteristics—the efficiency of its compressor, turbines and heat exchangers can be optimized to favour minimum fuel consumption at almost any specific operating point in the road-load range. Based on these factors, it was obvious that a study using specific fuel consumption as the criteria for decision would not be a realistic comparison of the diesel versus gas turbine.
Accordingly, it was decided to study various gas turbine cycles, and projected mid-1970-era diesel engine fuel consumption characteristics over simulated highway routes on the computer.
A 264-mile route from Detroit to Pittsburgh was selected, since a market study indicated that this route was representative of 50-per cent of all the inter-city tonnage transported in the United States. A longer route of 672 miles from Baltimore to Atlanta was also used as input, since analysis indicated that this route represented 33 per cent of the inter-city tonnage hauled.
A road profile of each of these interstate highway routes was programmed into the computer, consisting of measurements of distance and elevation at appropriately spaced intervals. In addition, the speed limits and the location of traffic lights were recorded.
The data were represented on the computer by a series of straight-line segments connecting each point taken in the road profile study. For each interval, the computer would first calculate the grade and the steady speed (either the selected speed or the maximum attainable speed, if lower). Knowing the condition at the end of the previous interval, it would cause the vehicle to accelerate or decelerate as required until either the steady speed was reached (in which case the interval would be completed at that speed), or until the total distance of that interval had been traversed, at which time a new interval would begin.
At each interval .on the computer it was possible to ascertain the exact operating conditions of the engine in regard to fuel economy, shaft speed, etc.; and for the vehicle, the rate of acceleration or deceleration, etc., and transmission gear selected.
The routes were proved out by actually operating vehicles with dynamometer-certified engine fuel consumption characteristics at various gross combination weights and average speeds. The viputer simulation was found to be extremely accurate—within 2 per cent of total fuel consumed.
With this degree of confidence, various gas turbine engine configurations were programmed for the computer and operated over the simulated highway routes. In all, 16 variations of gas turbine engines were studied at various vehicle gross combination weight and average-speed combinations. While the supercharged-cycle gas turbine appeared very competitive in routemiles-per-gallon throughout a wide operating range, the considerations of engine manufacturing cost swayed the decision to proceed with a simple, regenerative engine, using a ceramic regenerative heat-exchange system. In the final analysis of the selection, the basis for decision was the growth potential of this engine concept. The supercharged-cycle engine, for mechanical reasons, cannot use a ceramic regenerator. It is therefore limited in its design operating-cycle temperature—a feature which imposes a severe limit On its fuel economy potential. In other words, without an unforeseen breakthrough in recuperative heat exchanger design, the supercharged-cycle turbines studied represented the state of the art for the foreseeable future.
By contrast, an engine using a ceramic, rotary regenerator is limited only by the current state of high-temperature material technology. With the possible future advent of non-metallic materials for the turbine system, the simple, regenerative-cycle engine is the only one that would allow a future growth. The designer's choice the,n, obviously, has to be an engine with "built-in-growth potential".
From analysis to hardware The study and analysis described, as well as defining the engine cycle and component characteristics, optimized the engine power requirements. Fig. 11 illustrates the effect on average speed for the Detroit-to-Pittsburgh route, using various horse-power engines at 46.000 lb.gcw and 80,000 lb.gcw.
With the mid-1970 market in mind, a rating of 375 h.p. was selected. The engine which resulted from the analysis is designated the Model 707 and is shown in Fig. 12, installed in a production Ford W-1000 series highway tractor.
A cutaway, Fig. 13, illustrates the major hardware details-7-the airflow path and components are essentially identical to the cycle diagram in Fig. 1. By previous turbine engine standards this is a very simple, rugged powerplant; relying heavily on the use of low-cost cast components. Probably one of the most significant design features is the fact that a conventional manual 5-speed truck transmission and manually operated clutch is used. In fact, the durability of the transmission and clotch selected is a proven component in the United States' trucking industry. This was believed to be a strong requirement in the initial concept of the engine and vehicle drive-train. Acceptance of a turbine engine within the trucking industry would obviously be a difficult "teething': period. Compounding this problem with trying to get the trucker to accept an automatic transmission or unconventional driveline, together with a new engine concept, would probably be a formidable undertaking. In some respects, the combination of a gas turbine engine and manual transmission bring together most of the virtues of an automatic transmission without accepting the problems of lower driveline efficiency and poorer durability. The turbine vehicle can be "lugged down" in speed and even stopped in gear without _stalling the engine. The power turbine is a built-in torque converter, which provides excellent gradeability of the vehicle while using a minimum number of transmission ratios.
An additional feature of the 707 turbine is a built-in retarder. A .fourth pedal is provided on the floor of the cab (shown on the far left in Fig. 16). When negotiating a downgrade, the truck driver depresses the pedal and modulates retardation. Up to 250 h.p. is available down to approximately 15 mph, with no time limit on application. The retarder pedal is connected to the variable power turbine nozzles vanes, which actqate into the braking position when the retarder is depressed. The pedal also controls compressor turbine speed, which modulates the amount of retardation.
With an installed weight of 1,7001b—length 40in., height 39 in., and width at the frame rails of 28.5in., the powerplant is an attractive package.
Present status
It is now almost two years since the Ford Motor Company made an official announcement of the 707 truck engine development. In that period, four other world-wide automotive manufacturers have made similar press releases, or announced the development of truck gas turbine engines in the 300-400 h.p. class. All of these powerplants (with the exception of one manufacturer, using a recuperative-type heat exchanger) use the simple, regenerative-cycle concept. From this design trend and the fact that each of these engines has been based on the use of ceramic rotary regenerators, one could speculate that as the reciprocating-engine designers have gravitated toward the V-8 configuration of engine, the gas turbine designer has also pretty much "zeroed-in" on what could be the strongest competition for a truck diesel-engine powerplant.
, The question foremost in everyone's mind at this point is: -Where does the gas turbine truck engine stand?" And probably —the key question: "When?"
The Ford 707 engine is in an intensive period of development aimed at achieving the fuel consumption objectives and establishing durability. From the fuel economy viewpoint, the engine is at present very close to the on-highway fuel economy of the best truck diesel engines available. This in itself is a significant breakthrough in a low-cost, simple, gas turbine powerplant.
The initial durability objective of 100,000 miles without service is presently underway on a group of vehicles which, as well as being developed on Ford Motor Company's test tracks under simulated highway conditions are also operating in Company fleet service, hauling parts between assembly plants.
In parallel with track and highway development, extensive dynamometer testing is being carried out to achieve the ultimate durability potential of the engine. As the durability factor increases, Ford will seek the additional input of selected fleet operators by placing vehicles in highway service as a further part of the proving programme.
The financial consequences of introducing a new powerplant to industry, whether it be diesel or gas turbine, without the benefit of exhaustive proving, can be a financial catastrophe for both manufacturer and trucker alike. Therefore, the answer to: "When will gas turbines be offered for sale commercially in the trucking field?" cannot possibly be before the early 1970s; and then, only after the clear-cut economic advantage of this powerplant has been established.
The future—and impact on industry
There can be little question in the mind of any engine designer that the turbine engine possesses the potential to become the engine of the future; particularly considering the previously discussed developments in non-metallic materials which will allow turbine engines to be built with low-cost, hot flow-path components, operating at very high temperatures and efficiency levels. In recent years the development of inter-metallic and non-metallic materials has been a fast moving technology, due in some part to the requirements of the aero-space industry. Research and analysis performed by Ford Motor Company, resulting in the 707 engine development, has indicated that manufacturing cost, when compared with a diesel engine, may not be a deterring factor in production gas turbine manufacturing planning. The future, then, must be based on achieving significant fuel economy and durability improvement over projected mid-70 diesel engines.
Diesel engines, to some extent, are still a moving target for the gas turbine designer. The recent development and application of the high-torque-rise diesel engine in the United States is in some respects the diesel engineer's answer to the turbine engine. Combining a 5-speed transmission with a high-torque-rise diesel certainly takes some edge off the turbine engine's advantages. The fact still remains, however, that the diesel engine is being pushed hard from a durability aspect with each modification, whether it be turbocharging, compounding, or high torque rise. More significantly, its fuel economy or horsepower per cubic inch displacement has not improved by any large measure in recent years.
This, then, leads to the final observation of: "What could be the possible impact on the trucking industry in operating turbine vehicles and, more importantly, the maintenance aspect?" The operation of a turbine truck from the driver's viewpoint can be dismissed as a problem item. The Ford W-1000 turbine vehicles are no different to drive than their diesel counterpart. The operator starts the vehicle, engages gear arid shifts in an identical manner to that of a conventional vehicle. The only difference in driving habit is the requirement for less gear shifting.
From the maintenance aspect, the hardware is certainly very different; however, within.Ford Motor Company, this, too, has been an enlightening experience. It quickly became apparent that as the vehicles were moved from the research environment to test tracks for evaluation, both driver and mechanic personnel, who were unfamiliar with even the basic knowledge of how the engine operated, quickly adapted themselves to not only driving the vehicles, but performing service functions. In fact, within a very short period, these personnel were able to troubleshoot vehicles and perform fairly major engine teardown and overhaul with very little engineering guidance.
• To the fleet operator, the transition to turbine engine vehicles may not be too earth shaking. The turbine, once a mechanic has a basic knowledge of the engine, is no more sophisticated in hardware concept than today's diesel engine.
Conclusion
In conclusion, after almost two decades of development and, judging by the intense effort being expended by each of the automotive manufacturers active in gas turbine research, a highway truck application cannot be too far distant. Assessing the formidable competition that the turbine faces, it is probably safe to speculate that no manufacturer will risk a production venture without some tangible evidence of customer acceptance.
Acceptance can only be gained if the turbine can prove an economic advantage to the truck fleet operator. Therefore, it is probably safe to conjecture that, if the turbine does not revolutionize the trucking industry, it is certainly going to change its mode of operation dramatically.